Generation of ultrashort laser pulses
Written by: Mikhail Osolodkov
March 27, 2019
The interaction of light with atoms or molecules induces dynamics of their electrons. These dynamics naturally occur on the attosecond (10-18 s) timescale. In order to study such a fast phenomenon one needs a probe of comparable duration. Extreme ultraviolet (XUV) laser pulses meet this requirement. They can be generated using table-top laser systems through high-order harmonic generation (HHG) processes in different targets, such as atomic and molecular gases, liquids and solids. Generation of high order harmonics in noble gases (argon, krypton, neon, etc.) is the most investigated and well understood method. It is routinely used for a wide range of experiments in the field of ultrafast spectroscopy. The semi-classical three step model explains the mechanism of generation of laser pulses with hundreds of attosecond duration in such generation medium. Due to the importance of XUV attosecond pulses for the field of attosecond science in general and my research in particular I would like to discuss this model in more detail.
In our discussion we are going to use the single active electron (SAE) approximation. It assumes that only one electron is involved in the process. Also we assume the near infra red (NIR) laser field, for example with the central wavelength of 800 nm (10-9 m), to be relatively strong compared to the ionisation potential of the atom (the Keldysh parameter [1] is smaller than 1). Assuming this, the ionisation then occurs in the tunnelling regime. Keeping these assumptions in mind let’s go step by step through the HHG process.
The Coulomb electric potential of the atom is schematically illustrated in Figure 1. Without an external electric field (that corresponds to the phase of the field equal to 0) the electron occupies a particular state. In order to ionise the atom in the frame of classical mechanics (separate the electron from the ion and free the electron into continuum) it should absorb the amount of energy equal to or bigger than the ionisation potential (IP). When an electric field (for example an NIR laser field) interacts with an atom, it "bends" the Coulomb potential. As soon as the energy barrier is suppressed enough (close to the phase of the electric field equal π/2), tunnelling, a quantum mechanical phenomenon, starts playing a role. The probability for the electron to “escape” from the Coulomb potential to the continuum over this barrier becomes bigger than 0. An electron that has tunnelled through the barrier is no longer affected by the atom and moves as a “free” electron in the electric field. It is accelerated by the field until the phase becomes equal π, then it is slowed down around phase equaling 3π/2 and returned back to the parent ion around phase equaling 2π. One of the possible outcomes of the return of the electron to the parent ion is its recombination into the ground state. In that case all the kinetic energy that the electron has gained travelling in the field is released as a high energy XUV photon. Because the probability of recombination after return is very low, the number of generated XUV photons is extremely low. In order to optimise it, parameters such as density of the generation medium and intensity of the IR light in the interaction volume should be carefully adjusted.
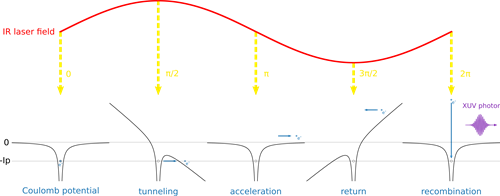
Figure 1: The schematic illustration the three step model (1 – tunnelling, 2 – acceleration and return, 3 – recombination) corresponding to different phases of the driving laser field.
In order to generate ultrashort XUV pulses the high order harmonic generation should be performed using short NIR laser pulses with tens of femtoseconds (10-15 s) duration. In Figure 2A a short NIR laser pulse is shown (the solid red line). Every time the electric field is strong enough to ionise the generation medium through the tunnelling mechanism described above (the ionisation threshold is shown as gray dash line) XUV photons are generated. In this particular case four separate bursts of XUV photons will appear and form an attosecond pulse train (APT). The interference of these bursts results in the formation of separated odd high-order harmonics of the fundamental frequency of the NIR light in the spectral domain. In other words, the spectrum of the XUV light consists of lines with energies equal to:
(2q+1)ħω,
where ħ is the reduced Planck constant, ω is the angular frequency of the driving NIR field and q is an integer. Only odd harmonics can be generated due to the reasons of symmetry (even orders are suppressed by destructive interference). In real experiments the APT consists of photons with energies equaling 15, 17, 19, etc. multiplied by the energy of the fundamental NIR photon.
Before moving to shorter IR pulses an important parameter, carrier-envelope phase (CEP), should be introduced. CEP is the difference in phase between the carrier wave and the intensity envelope of the laser pulse (in Figure 2 shown as solid and dashed red lines respectively). In Figure 2B and 2C few-cycle pulses with the same duration and π/2 CEP difference are shown. The interaction with the medium of the “cosine” pulse shown in 2B results in the generation of the isolated attosecond pulse (IAP). The spectrum of such a pulse is a broadband continuum. On the other hand the “sine” pulse shown in 2C generates a double XUV pulse which is not favourable for experimental applications. That's why the CEP stability of the few-cycle NIR laser pulses is essential for generation of isolated attosecond pulses. For more information about generation of ultrashort laser pulses, their properties and applications in attosecond science please refer to Ref. [2].
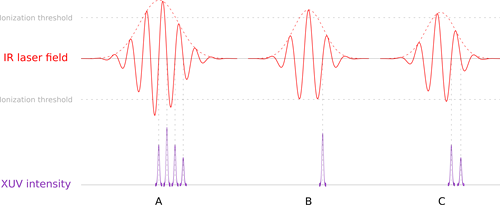
Figure 2: The schematic illustration of the temporal profiles of the XUV pulses generated by the IR laser pulses with different duration and carrier-envelope phases. A – attosecond pulse train, B and C – isolated attosecond pulse and double pulse generated by “cos” and “sin” IR pulses with the same duration respectively.
Our experiment
In our experiment we are planning to investigate ultrafast electron dynamics that follow the photoionisation in the diatomic molecules such as H2, N2, CO, etc. using COLTRIMS technique [3]. Coincidence detection of photoelectrons and fragment ions will allow us to investigate this phenomenon in the molecular frame. The few-cycle NIR pulses delivered by high repetition rate (100kHz) non-collinear optical parametric chirped pulse amplification system (NOPCPA) are used to generate isolated attosecond pulses, which are necessary for our experiment in order to achieve required temporal resolution. The final improvements of our setup were just completed and we are ready to start the proposed experiments in the nearest future.
Personal experience as ESR in ASPIRE
As an Early Stage Researcher participating in ASPIRE International Training Network I am provided with an exclusive opportunity not only to work at the state-of-the-art experimental setup, such as one I am working presently at Max-Born-Institute in Berlin, but also to participate in its development and improvement. The exchange of knowledge inside our research group and through the Training Network helps me efficiently learn many different skills necessary for a scientist nowadays. On the other hand, being in permanent connection with the leading research groups in the field all over the Europe provides the possibility to be well informed about new developments and discoveries that is, in my opinion, essential for successful research.
References:
[1] Constant Schoulder ASPIRE Blog article
[2] Ferenc Krausz and Misha Ivanov, 2009, Rev. of Modern Phys., 81
[3] Giammarco Nalin ASPIRE Blog article